Plate Tectonics
A Dynamic Planet
When we look at a map of the earth, we see that its political boundaries have changed over time as kingdoms and nations have risen, changed, and disappeared. Yet over thousands of years of history, the shape of the continents and the position of the oceans have remained largely the same. We can imagine that it has always been so. But a key conclusion from about 150 years of scientific observations of the earth is that this is not the case. On the surface of the earth, continents are in motion relative to one another and the oceans are also changing in size and shape. The world map of the distant past looked nothing like that of today, and the world map of the distant future will likely appear far different as well. While on a daily, yearly, or even millennial scale things appear the same, on a geologic scale of millions and billions of years, we live on a dynamic planet.
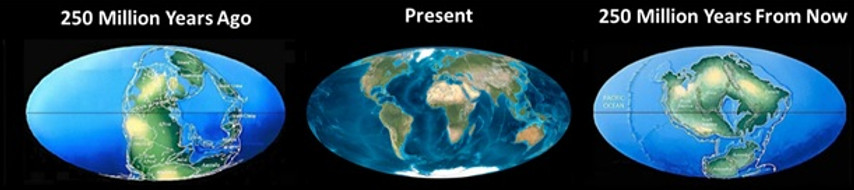
Map images from: NASA (left and right images); The Australian Museum (center image).
Since the completion of the first accurate maps of the world, a number of scholars had noted the similarity of the shapes of continents on both sides of the Atlantic and that these might fit together if the Atlantic Ocean were removed. In 1596, the cartographer Abraham Ortelius suggested in his work Thesaurus Geographicus that the Americas had been "torn away from Europe and Africa . . . by earthquakes and floods" and went on to say: "The vestiges of the rupture reveal themselves, if someone brings forward a map of the world and considers carefully the coasts of the three [continents]." (Kious and Tilling 1996).
​
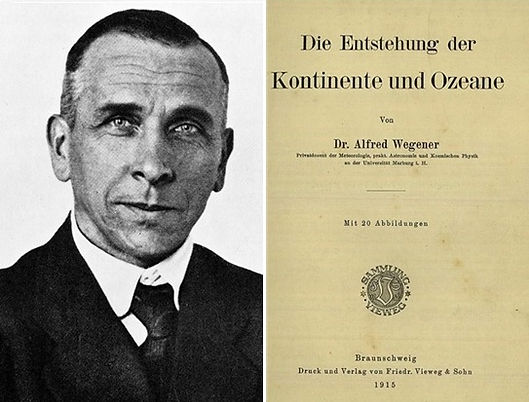
This insight on the similarities of continental coastlines cropped up in scholarly writing sporadically, but it was not until the early 20th century that the German meteorologist and geophysicist Alfred Wegener, put forth a fully formed hypothesis of continental displacement, arguing that continents moved relative to one another and that about 250 million years ago all the continents were joined together in a giant supercontinent he called Pangea. In spite of his recitation of diverse data from geography, geology, and fossil evidence, Wegener was largely derided by the scientific community for this idea, mainly because there did not appear to be a convincing mechanism for how the continents could move in such a way. He died during an expedition to Greenland in 1930 seeking support for this theory, and his hypothesis of continental displacement remained largely ignored over the subsequent decades. Wegener’s rejected hypothesis reminds us of a quote from another German, Albert Einstein: “If at first the idea is not absurd, then there is no hope for it.”
Left: Alfred Wegener. Right: A 1915 edition of his book, Die Entstehung der Kontinente und Ozeane (The Origin of Continents and Oceans). Image sources: Left: E.Kuhlbrodt - Bildarchiv Foto Marburg Aufnahme-Nr. 426.294, Public Domain, https://commons.wikimedia.org/w/index.php?curid=18166661; Right: Ketterer Kunst.
Key Principals of Plate Tectonics
​
1. The Heat Engine of the Earth
​
The key to plate tectonics is what we can call the heat engine of the earth. The “work” of this engine is fueled by heat radiating from the center of the earth, at which lies a core of white-hot metal—mainly nickel and iron but also other elements. The core temperature is estimated to be about 6,000° F (10,800° C): hotter than the surface of the sun. Normally, a metal mixture at this temperature would be entirely liquid, but the pressure at the center of the earth is so great that only in the inner part of the core—a diameter of about 1,500 miles—is this mixture of metal in a molten state. At around 750 miles up from the center of the earth, the metal mixture transitions to a solid form. This solid but superhot outer core extends another 1400 miles up. The circulation of liquid metal at the very core of the earth is responsible for generating the magnetic field that protects the planet and helped make life possible. However, the circulation within the core is dynamic. One result of this circulation is that the location of “magnetic north” changes from time to time—in 2019 it dramatically shifted by several hundred miles—and every few million years the magnetic poles reverse, so that “north” is at the south pole end and “south” is at the north pole end.
​
This largely metal core is covered by a rocky layer about 1800 miles in thickness called the mantle. The mantle is composed of rocks of magnesium and iron silicates of high density. Recent research has suggested that in this lower region are large areas of liquid that are geochemically distinct from the solid material.
Using remote techniques such as measuring the behavior of pressure waves generated by earthquakes, scientists have identified a transition that occurs in the mantle about 410 miles below the earth’s surface; this likely marks a change in the overall chemical composition of the rock. This upper 410 miles of mantle is called the upper mantle and it is a place where the heat rising from the core is translated into movement at the surface. About 250 miles below the surface and with a thickness of about 170 miles is the asthenosphere. This is where the dynamics that move the earth’s surface are generated. While the asthenosphere is composed of rock like other parts of the mantle, the effects of heat and pressure make asthenosphere plastic and ductile; some regions of the asthenosphere are molten (liquid rock). It is likely that the lower mantle does not possess the same plasticity as the upper mantle because the increased pressure on the rocks at that depth keep them in a solid state.
Between 80 and 20 miles in depth, the decreased pressure and temperature makes the mantle much more rigid. This zone of the mantle is called the lithosphere. Riding atop the lithosphere is the crust. The basaltic ocean crust has a depth of 3-6 miles while the continental masses can be up to 20-25 miles thick. The figure to the right shows these layers of the earth.

Here is how the heat engine of the earth produces movement on the seemingly solid surface. The tremendous heat at the core of the earth is continuously radiating up through the layers of the earth. If the earth was a solid mass of uniform composition, the conduction of this heat would be uniform. About 100 miles down the temperature and pressure are sufficient to make rock behave in a more plastic manner—able to change and flow at very slow rates. Under these conditions, the radiation of heat from below creates a series of convection cells within the earth, with regions where mantle carries heat upward and other areas where cooling mantle material descends. You can see this dynamic in a “lava lamp” where heat at the bottom of the lamp causes the portion in the center to rise and, as it cools, to then descend.
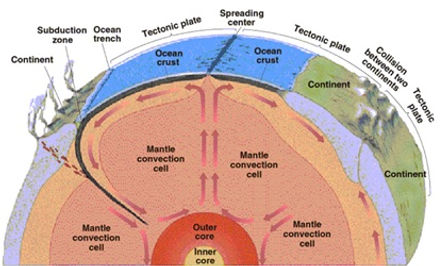
Image source: University of Syndey, Australia
The area of rising hot mantle in the convection cell carries heat upward to the crust and focuses it, resulting in melting of the rock above. Hot liquid rock is lighter than the solid rock around it, and this liquid rock—called magma—makes its way toward the surface. If the convection zone forms a vertical plane, ocean ridges are created where the hot magma from below is expelled to form new oceanic crust. The formation of new crust will continue as long as it is fed by the convection of the mantle below. Once this stops, the creation of new ocean floor also stops.
In some cases, the convection zone is a more focused spot and creates a magma plume or “hot spot” under the crust instead. A small plume may form oceanic volcanoes like those of Hawaii. As the oceanic crust moves above the hot spot, new volcanoes gradually form while older ones become extinct and erode into the sea. A magma plume on a larger scale can initiate the development of new spreading zones and lead to the breakup of assemblies of continents, as we shall see for Avalonia.
As the convection circulation meets the solid rock above and spreads out, it pushes the plates above it apart from the ridge. Early on it was through this push was the driving force in plate movement, but recently, scientists have concluded that the gravitational effect is more crucial. First, in the areas where the mantle is pushing up cause a bulging of the earth that creates gravitational differences. The solid ocean crust tends to “slide” toward the area of lower gravity. When one plate moves under another this gravitational difference is accentuate. Plate tectonics is due not only to "ridge push" but chiefly from "slab pull" and these forces may be different in different plates. The circulation of hot mantle, fed by the tremendous heat from within the earth, therefore becomes the engine that drives the movement of the oceanic plates and the continents. The rate of movement of the mantle and the plates above it are very slow—typical rates of sea floor spreading are only about an inch per year (in some cases this may be much as 5 times faster). Yet over millions of years this can move the overlying crust quite far.
​
Another key to plate tectonics is that these convection cells are not constant. New circulation patterns can appear over time leading to the formation of new plate margins, while the cessation of older cells results in the stoppage of sea floor expansion.
​
2. The Dance of the Continents
The dynamic of “sea floor spreading” that creates new oceanic crust presents a fundamental problem –what happens to the rest of the crust? Where does it end up? Early on in the development of plate tectonic theory, one scientist hypothesized that the earth was gradually expanding in size as a result of this process. But this is not the case. Instead, at some point the oceanic crust will meet another portion of crust or a continental mass moving in a different direction. Something has to give! Typically, one portion moves beneath another. The portion of plate sliding under the other is called subduction. At the subduction zone, the crust is pushed deeper and deeper into the earth—not easily, as this process frequently results in earthquakes as the resistance to this movement suddenly breaks free—until it encounters the deep, hot mantle. At this point the plate is melted and recycled deep in the earth. In simplistic terms, the heat engine of the earth, transmitted through the mantle, results in moving plates at the surface that spread from a hot mantle zone and are pushed down back into the mantle at a distant end—a very slow and persistent conveyer belt of rock.
As the crust is subducted many miles below, a combination of heat within the mantle and water pulled down with the crustal slab produces melting of the crustal plate. This melted rock—magma—begins to rise (liquid rock is less dense than solid rock) through the overlying rock. It may melt some of the continental rock to produce a new mix of rock. This magma may be extruded at the surface through cracks or from the formation of volcanoes. Or it may solidify underneath the surface in bodies called plutons (really large plutons are called basoliths, which is good to know for your next dinner party).
In some cases oceanic crust meets, not other chunks or plates of oceanic crust, but continental masses instead. Unlike oceanic crust, which is largely composed of a heavy dark rock called basalt (the rocks of the mantle are very dense), continental crust is composed of much lighter materials, typically derivatives of granite. When heavier oceanic crust meets lighter continental rock, the oceanic crust will usually move underneath, creating a subduction zone just off the continental margin. The figure below shows two different types of subduction zones: one where ocean is pushed beneath oceanic plate (left) and one where an oceanic plate is being subducted beneath a crustal plate.

Image from: University of Sasketchewan
In some cases different plates may move by each other. This sideways motion, which is classically found in the San Andreas fault, results in extensive earthquake activity over time, but volcanism is minimal compared to subduction. This fact is important to our story about Avalonia. Finally, there is evidence that when oceanic crust attached to continental crust is subducted below other ocean crust, when the oceanic crust is entirely subducted the other oceanic plate can "run up" onto the continental plate. This condition is called obduction. Obduction leaves some evidence with unusual forms of igneous rock (the formations are called ophiolites).
In the Pacific Ocean we see all the dynamics of plate tectonics at the same time (although not obduction). There are large sea ridges where ocean crust is generated and long trenches where sea floor dives below continents. There are also hot spots that form a series of high volcanoes. Some of these become large enough to rise above sea level. The Hawaiian islands are an example of this. However, if one looks at a topographic map of the Pacific, the trail of past (now dormant) volcanic mounts can clearly be seen.
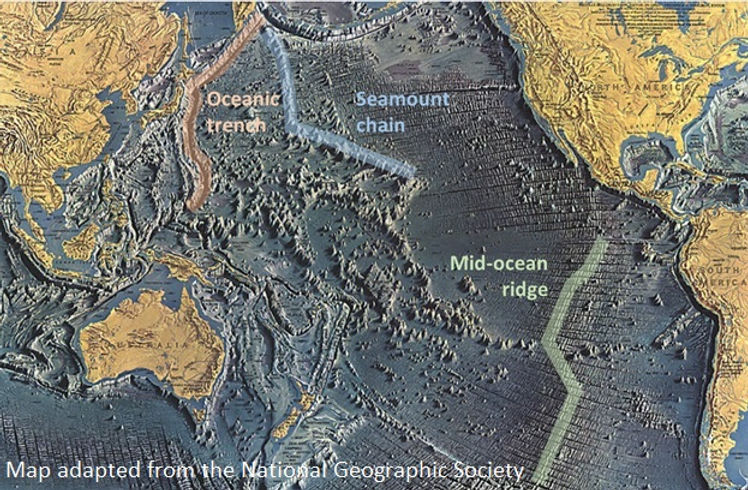
Heated by the core within, the mantle demonstrates a circulation of heat in a series of cells. In some areas, heated mantle material is ascending. In other areas, cooling material is descending. Plate boundaries form along these areas of ascending mantle and heat release. This heat causes melting of the crustal material, which then rises as liquid to the surface along ocean ridges. In most cases this happens far below the surface of the water, but in one place—Iceland—the ridges have built to a level above the water, and eruptions of new crustal material occur periodically. Scientists have currently identified seven major plates along with nine other smaller plates. This number is not constant over time, with new plates created and older ones completely subducted and recycled within the earth. The continental masses are too light to be pushed down into the mantle (think of pushing a cork under the water), but the continental masses are pushed together and pulled apart over time.
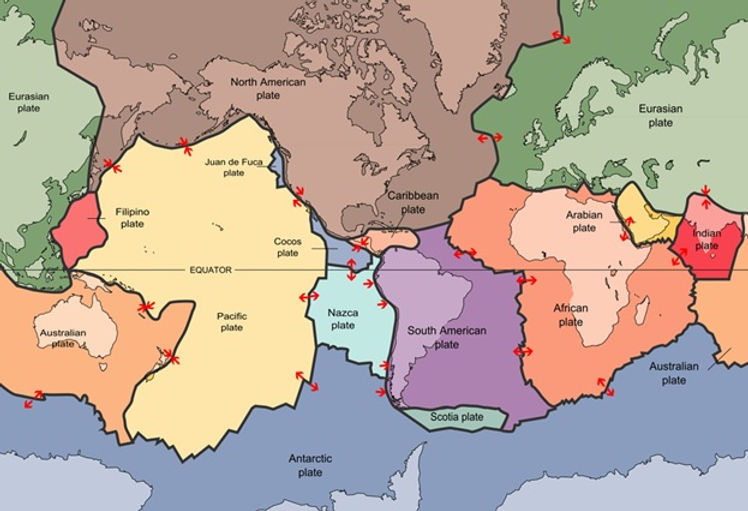