The Avalonian Rocks of the Boston Basin and Their Ancient Depositional Environment
To see some samples of rocks discussed in this page, click here

Avalonian terrane makes up a large portion of eastern Massachusetts but is not the only microcontinent terrane found in the northeastern part of North America, because Avalonia was not the only ancient microcontinent that made a journey from the edge of Gondwana to Laurentia (more on that here). Ganderia, which was accreted to Laurentia before Avalonia, formed off the edge of the Amazonian craton rather than in the ocean like Avalonia. Ganderia and Avalonia were geographically in proximity to each other when they were rifted away from Gondwana by the opening of the Rheic Ocean. Ganderia’s accretion to Laurentia happened with the closing of the Iaepetus Ocean and resulted in a significant mountain building event, the Salinian orogen. When Avalonian contacted Ganderian terrane, it was thrust under it; the contact point between Avalonian terrane and Ganderian (Nashoba) terrane is clearly demarcated in Massachusetts by the Bloody Bluff fault (see Figure at left). Like much of New England, the bedrock of Avalonia is extensively igneous or metamorphosed igneous rock.
Figure adapted from: Minute Man National Historical ParkGeologic Resources Inventory Report. Natural Resource Report NPS/NRSS/GRD/NRR—2017/1523. National Park Services.
Some of bedrock in the Avalonian terrane was formed much later after the initial formation of the island arc. As can be seen from the figure above, while the main period of Avalonian formations found in eastern Massachusetts date from its volcanic arc phase (Neoproterozoic), a number of Paleozoic igneous rocks are found in Avalonian terrane, including the Cape Ann Pluton, the Peabody Pluton and the Quincy Pluton. These were formed during the accretion of Avalonia to Ganderia. The composition of the igneous rocks in these plutons tell the story of the melting of continental crust, not the oceanic crust that characterized magmatism in Avalonia during its formation. Within the Avalonian terrane is also the Narragansett Basin, a sedimentary deposit dated to Permian time, which contains erosional deposits from the mountains built when Avalonia was being compressed between Laurentia and Gondwana during the formation of Pangea (Act 7).
The general layout of the Boston Basin (figure below) is a fault-bounded sedimentary basin surrounded by igneous and metamorphosed igneous rocks. Basin rocks have undergone modification with the collision of Avalonia with Laurentia and the subsequent collision that formed Pangea. Some of the strata are tilted and folded, with the axis of one of many of these folds running southwest to northeast. One of these runs through the Brookline area (see the trend line within the red Brookline outline in the Figure below), roughly paralleling route 9 on the south side of the Brookline Reservoir. The sedimentary rocks have also been modified by heat and stress, resulting the development of cleavage within some rocks and an unusual density and structural strength to the conglomerate. As a result, it has been used as a building stone in many well-known Boston churches and other landmarks and has been designated the official Massachusetts state stone. Mudstones and sandstones deposited within the conglomerate were modified by the same forces, with some of the mudstones having a slaty appearance.
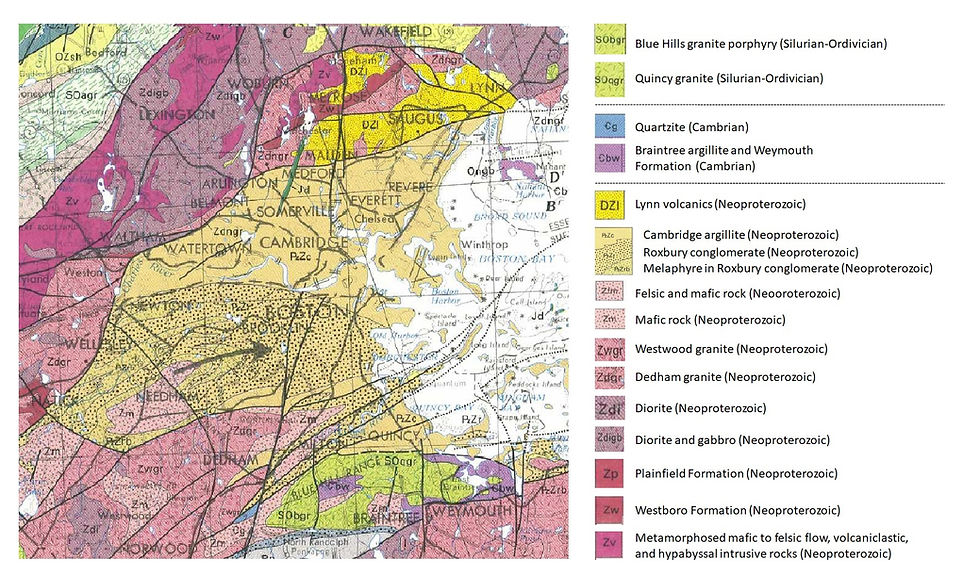
Figure: Close up of the bedrock map of Massachusetts showing rocks of the Boston area. Key ranks rock types in order of age (youngest on top). Tan colors show sedimentary and igneous rocks of the Boston Basin. Most igneous and metamorphosed igneous rock surrounding are from the Neoproterozoic and of Avalonian origin; the Blue Ridge Range rocks are distinctly different, representing magmatic intrusions into Avalonian rock about 440 Ma. Image adapted from: Zen, E-an, Goldsmith, R, Ratcliffe, NM, Robinson, P, Stanley, RS, Hatch, NL, Shride, AF, Weed, EGA, Wones, DR. Bedrock Geologic Map of Massachusetts. US Geological Survey, 1983.
A bedrock geologic map developed by Thompson et al. 2014 (figure left below) provides more detail on the Avalonian rocks of the Boston basin. The red outline in the map shows the boundaries of Brookline. The rock formations within the Boston basin mark the transition of this part of Avalonia from subduction-island arc magmatism (Act 3) to transform faulting-rifting magmatism and to passive margin sedimentation (Act 4). The Boston basin may originally have formed from shear stress exerted on the Avalonian island arc during subduction, as the angle of subduction was relatively low, exerting significant transverse stress on the arc margin (Figure right below). The combination of this subduction and the shear stress could have initiated pull-apart basins in the arc.
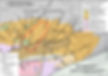

During Avalonia's magmatic arc period (around 635-590 Ma), subduction at the forearc was accompied by significant shear stress caused by plate movement at a oblique to the island arc (see arrows showing direction of plate movement), Location of Brookline shown in red dot. Figure adaptred from: Nance et al. 2012.
Figure from: Thompson et al. 2014. Reprinted by permission of the American Journal of Science.
Geochronology of the Avalonian Rocks of the Boston Basin
The rocks of the Boston Basin are a repository of a specific period in Avalonia, during the time it made a transition from an active volcanic arc to a passive arc platform. The period of time these rocks cover is about 610 Ma to 540 Ma (Neoproterozoic--Early Cambrian). The figure below shows the time period for deposit of these different rocks. Ages have been established through a variety of isotopic techniques appropriate to rocks of this age. The summary below covers these rocks in chronological order of their formation.
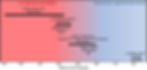
Dedham Granite (630-596 Ma): The oldest rock in the Boston basin, it is an intrusive igneous rock that reflects the melted crust from the subduction margin. The deposit located at Hyde Park was dated at 609 Ma. Dedham Granites to the south and west are older, dating back to 630 Ma, while Dedham Granites to the north of the Boston basin are slightly younger at around 596 Ma, reflecting continued arc magmatism during the time the sediments began to be deposited in the basin.
Lynn-Mattapan Volcanics (602-593 Ma): In contrast to the intrusive Dedham Granite, the Lynn-Mattapan Volcanics are extrusive, resulting from eruptions of lava and ash. Like the Dedham granites, this igneous complex brackets the Boston basin, in Mattapan to the south and Lynn to the north. These date somewhat younger than the Dedham Granite, with the Mattapan portion dating to about 593 Ma. Intrusive basalt dikes and sills within both the Dedham Granite and the Westwood Granite may be part of the Mattapan Volcanics.
Westwood Granite (599 Ma): Dated earlier at 579 Ma and more recently at 599 Ma, this igneous rock may be the intrusive equivalent of the Lynn-Mattapan volcanic complex.
Roxbury Conglomerate (595-580 Ma): Within the boundaries of Brookline, most of the bedrock found at the surface belongs to what is called the Roxbury Conglomerate, dating between 595-585 Ma (see below for exception). These rocks are mainly composed of metamorphosed sediments that collected in the Boston basin from erosion of the Avalonian island arc built up by subduction during the time Avalonia was attached to Gondwana. The Roxbury Conglomerate contains a mix of conglomerates, sandstones and mudstones, but is dominated by conglomerates. Clasts within the conglomerate range widely in size, with some formation containing boulder-sized rocks and others mere pebbles. In general, the size of the clasts within the conglomerate decrease in size from south to north and a number of geological indicators suggest the original slope of deposition was in a northern direction (based on current orientation). In addition, the clasts within the conglomerate are often from the nearby igneous or metamorphic source rocks. For example, Roxbury Conglomerate outcrops in the south and southeast part of the Boston basin contain clasts eroded from the Dedham Granite, while conglomerates of the north contain portions of the Westwood granite. Conglomerates from the north show evidence of quartzite inclusions derived from the Westboro quartzite up in the Middlesex Fells.
Four distinct subunits have traditionally been identified within the Roxbury Conglomerate. The Franklin Member is considered the oldest, then the Brookline, Dorchester and Squantum Members. Neither the Dorchester Member or the Squantum Member appear in the Brookline area. The Franklin park member is dominated by relatively unsorted conglomerates with very few instances of sandstone or mudstone interbedding. The conglomerate clasts are of highly variable size, including some very large cobbles, compared to conglomerates found in other areas; in general, these clasts may be less rounded. Both the inclusion of large clasts and the degree of rounding suggest deposition closer to the source of erosion.
The Brookline Member makes up the majority of Roxbury Conglomerate outcrops in the Boston Basin, and the majority of the outcrops in the northern part of Brookline. The classic “Roxbury puddingstone,” it contains conglomerate with clasts of modest size interbedded with sandstones and mudstones. Many outcrops show evidence of marine sedimentation, particularly as part of turbidites, but there are areas that also appear to show evidence of land-based (stream or river) deposition. The age of zircons found in the clasts of the conglomerate constrain the maximum age of deposition to about 598 Ma , while deposits of Brighton Igneous rock intruding and capping this rock restricts the youngest age to about 584 Ma.
The Dorchester Member is largely composed of purplish, greenish and grey siltstone, sandstone, and medium-to fine-grained argillite (a hard mudstone). It contains lesser amounts of sandstone and conglomerate than the Brookline Member and shows characteristics of marine turbidite deposition. In the latest geological map of the Newton Quadrangle, Dr. Margaret Thompson does not make a distinction between Dorchester and Brookline Members. Dorchester deposits may represent deposits much further from the source of erosion, perhaps in a deeper portion of the basin.
The Squantum Member outcrops in the southeastern part of the Boston Basin, particularly along the Harbor islands and the south shore, including Hingham. The Squantum “Tillite” is famous for its early characterization as evidence of being glacially deposited (and later as a piece of evidence for what is called the “snowball earth” hypothesis) but many other analyses have undermined this hypothesis and have attributed deposition to a similar marine deposition that characterizes much of the other Roxbury Conglomerate members (see for example Carto and Eyles 2012). The upper age of the Squantum Member is constrained at about 595 Ma based on the dating of an interbedded tuff and the youngest age of zircons in igneous clasts within the sediment. Using a recent geochronology reconstruction of the Boston Basin by Thompson and Crowley (2020), I would suggest that the Squantum Member may record a period of deposition that may have occurred at the cessation of magmatic activity in the Boston Basin but in conjunction with the Gaskiers glaciation. Changes in sea level and the erosive effects of ice may have triggered this depositional effect. In such a case the deposit may date to around 580 Ma making this rock the youngest in the Roxbury Conglomerate. Others see the evidence pointing to sedimentation in the context of local glaciation (Passchier and Erukanure 2010), which is consistent with the polar location of Avalonia during this period.
The Roxbury Conglomerates have been modified through subsequent metamorphism during the initial accretion of Avalonia to Laurentia and the following compression with the closing of the Rheic Ocean and formation of Pangea and can thus be considered a metasedimentary rock.
Brighton Igneous Suite (585 Ma): Within the Brookline Member, a set of intrusive and extrusive igneous rocks called the Brighton Igneous Suite can be found. These were either intruded into or erupted atop existing Roxbury Conglomerate sediments (or both). Some of these rocks show evidence of being deposited underwater. An example of the Brighton Igneous Suite in Brookline can be seen in Dane Park in Brookline, where the rocks have been dated to 584 Ma (Thompson et al. 2014). The Brighton Igneous Suite is much more extensive on the western border of the Boston basin, with a thickness of over 2,100 m (about 6,900 feet).
The period of deposit for most of the Roxbury Conglomerate was at the transition between the magmatic arc period of tectonic activity in Avalonia and the transition from subduction to transformational faulting as the spreading center that was feeding oceanic plate material into the subduction zone came into contact with the subduction zone, largely ending island arc volcanism. Both the subduction, which occurred at a low angle to Avalonia, as well as the subsequent transform fault movement, exerted significant stress on the back arc region, creating faulting, rifts and basin formation, and resulting in the deposition of intrusive and extrusive igneous rocks. The Brighton Igneous Suite may have resulted from these types of tectonic processes. Thompson et al. (2014) attributes the Brighton Igneous to magmatic arc activity.
Cambridge Formation (560-540 Ma): Also referred to as Cambridge Argillite, this is a fine-grained mudstone that is in parts massively bedded. Portions contain fine sandstones as well. The thickness of the Cambridge Formation is estimated to be about 4-6 km (2.5-3.8 miles) and to have been deposited over about 25 million years. The deposit in the Boston Basin was first radioisotopically dated to a maximum age of 570 Ma from uranium-lead dating of zircons found in an ash bed deposited at the base of the formation found in Somerville (Thompson et al. 2000). In addition, the fossil Aspidella, a Neoproterozoic organism typically found in deep water fine sediments, and which was identified in Cambridge Argillite during tunnel construction near Harvard Square, has been dated to between 610-550 Ma. This formation has been interpreted as being deposited in a deeper water, shelf-slope-basin environment, and may have been located within a deeper rift- or wrench-basin (Bailey 2013). Some investigators considered it to be roughly contemporaneous with the Roxbury Conglomerate and represented the distant portion of this formation. Although the geographic configuration of the Cambridge Formation makes this a possibility, the discontinuity between the two formations (mainly seen in tunnel deposits and rock cores) has been problematic. Recent excellent work by Thompson and Crowley (2020) has provided a better explanation. Their work includes an important revision of the dating of the ash bed deposit to about 550 Ma, suggesting that the Cambridge Formation formed much later than the adjacent Roxbury Conglomerate and likely represents a shelf formation deposited following the transition of this portion of Avalonia from an island arc at the edge of an active subduction zone to one with a quiescent transform margin. It is possible that during the closing of Pangea, some portion of the Cambridge Formation was thrust over parts of the Roxbury Conglomerate.
For the most part, the Cambridge Formation is not exposed on the surface in Boston. Examples of it can be found in parts of Somerville and Arlington to the north and Dorchester and Quincy to the south, especially in the harbor.
Depositional Environment
The sedimentary rocks of the southern part of the Boston Basin are dominated by conglomerates with clasts of widely variable size. Many of them are poorly sorted and large cobbles may be embedded in a matrix of fine sand or mud. Early on many geologists posited that they were likely deposited in a glacial environment, where melting ice released these clasts into a mud or sand matrix. However, bedding structures within the deposits, along with the interbedding of fine sand and mudstone, suggest that they were deposited in a near shore marine environment, and that many of these deposits can be classified as turbidites. Turbidites are formed by the slumping of deposited sediments in underwater environments. Instability along steep slopes results in underground “slides” that can scour the seabed, depositing new sediment. Often, large clasts and smaller fine matrix can slide together, forming jumbled assortments.
The geographic position of each of these rock units, with the older, igneous units surrounding the younger sedimentary units within the basin suggest that the Boston basin was a subsistence basin formed during the time of magmatic arc activity around 600 Ma. After subsistence, it formed a steep slope for the collection of erosional products from the surrounding igneous sources. The initial sedimentation was proximal to the rock origin and deposited upon steep slopes in the form of debris flows. Over time, the slope angle of erosion decreased and the distance of transport increased, allowing for more sorting of sediments, resulting in alternation of conglomerates, sandstones and siltstones depending on the depositional context. At the distal end, deposition was mostly in the form of turbidites and siltstones. Some portion of the basin was underwater at times, and there is evidence from geochemical changes in these rocks that it had some exposure to the open sea early on (Socci and Smith 1987). The timing and nature of the deposits suggest volcanic and sedimentation activity that occurred in the context of magmatic arc activity prior to the quiescent period brough on with a shift from subduction to transform faulting. Most recently, Galli and Bailey (2018) conclude that the igneous and sedimentary rocks within the basin are consistent with a rift or wrench basin formed in the late stages of development of a subduction/magmatic arc system associated with continental basement.
In general, across the conglomerates in the Boston basin, there is trend of larger to smaller clasts within the conglomerates as one moves from south to north. This suggests that rocks deposited in the (now) southern area were closer to the source rock than those further to the north, implying the opening of the basin was generally northeast. A nice illustration of what the depositional environment for the Boston basin may have been like has been provided by Galli and Bailey in their 2018 review (Figure below left). This picture is significantly different from the first hypotheses raised for the formation of these rocks in the early 20th century or in the decades after the consensus around plate tectonic theory. This is due to the continued field work of a number of geologists, along with improvements in tools for evaluating radiometric dates and analysis of the geochemistry of samples. As additional work is done and discoveries are made, this picture may be further defined or modified.

Figure: Schematic of Boston Bay depositional environment. In such a rugged basin a major fall in sea level exposes shelf and upper slope deposits and destabilizes portions of the outer shelf and upper slope. Rivers incise into the exposed shelf and upper slope and deliver coarse clastics directly into the deeper basin by an array of sediment gravity mass flow transport mechanisms. Figure from:Galli and Bailey 2018.
While early on, a leading hypothesis regarding formation of the Roxbury Conglomerate posited that it was deposited as a result of glacial activity, subsequent work over the last several decades has not supported this hypothesis, although some investigators ascribe the deposition of the Squantum Member to glacial influences (e.g., Passchier and Erukanure 2010). Nevertheless, regional or global cycles of glaciation that may have occurred over the period of deposition for the Roxbury Conglomerate, such as the Gaskiers glaciation, would have significantly altered sea level, and such changes would have changed the environment in which some of the Boston basin deposits occurred. The red boxes in the figure to the left show that the type of sedimentary deposits in the same area can be significantly different based on the sea level at the time of deposition.
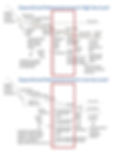
Figure: How Sea Level Change Alters Depositional Environment. Figure adapted from: Galli and Bailey 2018.